Research
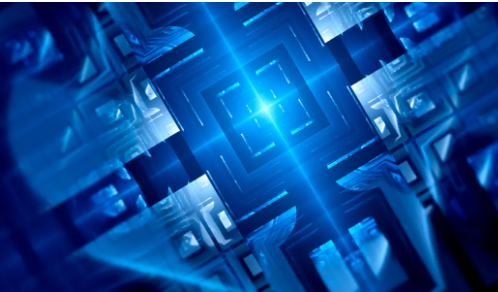
Empowering Practical Interfacing of Quantum Computing (EPIQC)
One of the main challenges to scale quantum computing technologies is how to efficiently address large numbers of cryogenic devices. Fully fledged quantum computers will require control interfaces capable of reading and manipulating millions of qubits operating down to millikelvin temperatures. This mighty technical challenge is shifting the research focus from the physics of individual qubits to engineering and systems integration. Avenues to explore include the design of dense and modular control channels, enhancements in signal bandwidth, in time and amplitude resolution of generated waveforms, as well as efficient power management of cryogenic control electronics.
Project EPIQC will address these issues through three complementary approaches: optical interconnects, wireless control/readout, cryogenic integrated electronics. Through a collaboration between ICT experts and quantum hardware specialists, EPIQC will validate novel multi-qubit interfaces across a range of quantum computing platforms, such as superconductor and semiconductor devices. This work is part of a collaboration among University of Strathclyde, University of Glasgow, National Physical Laboratory, National Quantum Computing Centre and University College London.
Funding :
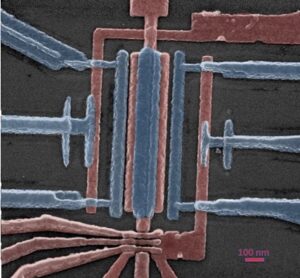
Silicon Quantum Devices
Silicon is the cornerstone of the digital age. Improvements in the processing of this semiconductor have led to relentless miniaturisation of integrated electronics and to a spectacular increase in computational performance. We work on nanoscale silicon transistors to turn them into quantum mechanical devices (e.g. quantum dots) for applications in quantum computing and quantum metrology. We are developing technology to shuttle individual electrons, read and control their spins, as well as interfacing quantum and classical control electronics. We contribute to the efforts of the National Quantum Technology Programme through synergies at UCL and NPL.
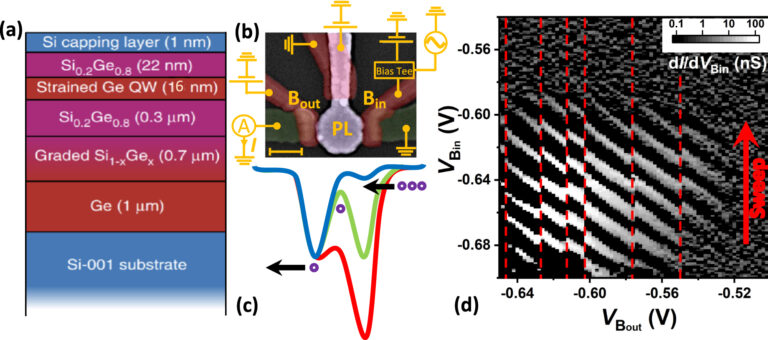
Germanium Hole Pumps
Not many people know that the very first transistor developed by Bell Labs in the 40s was made of germanium. Today this material is still widely used for high frequency electronics, particularly for telecommunications purposes. We use Ge quantum dots to realise single-hole pumps, a type of quantum device that could become a metrological tool for the realisation of a quantum-based standard of the unit of electric current, the ampere. We collaborate with NPL and TU Delft to characterise and manufacture these systems.
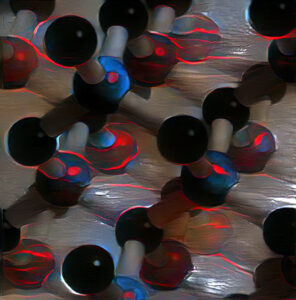
Quantum Electronics In Silicon Carbide (QELTIC)
Silicon Carbide is a semiconductor widely deployed for applications in high-power electronics for its resilience against large electric fields and elevated temperatures. Lately, SiC has emerged as a promising material for quantum applications too. This is because it hosts quantum effects that can be exploited to build a range of useful devices ranging from environmental sensors to secure communication devices and enhanced computing apparatuses. We aim to develop the underpinning technology to realise the first generation of quantum nano-devices in SiC that can control spin, charge and photonic degrees of freedom entirely electrically. In this endeavour, we closely collaborate with NPL, Hitachi and BT.
Funding:
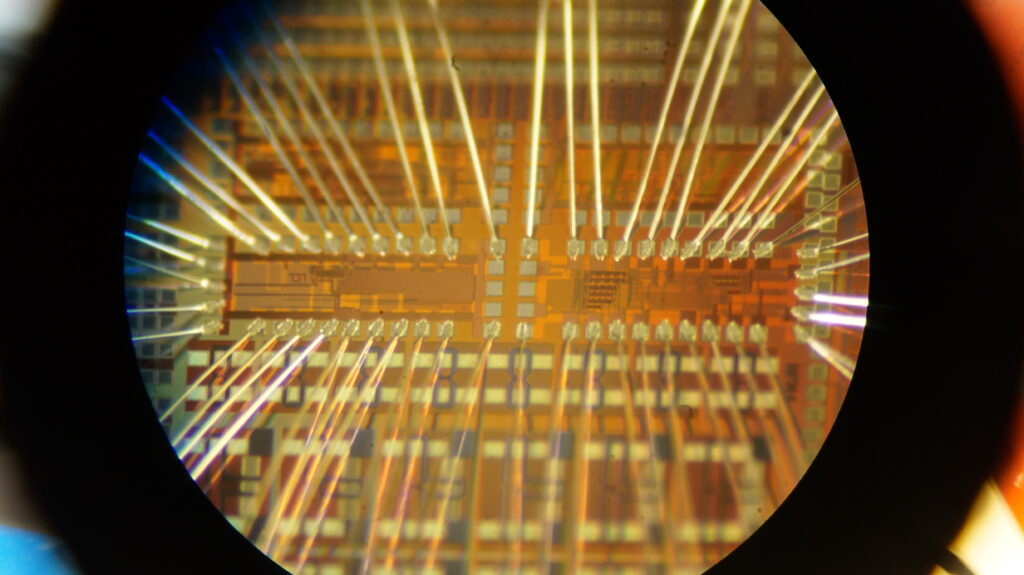
Cryoelectronics For Quantum Circuits (ALTNAHARRA)
In order to develop large-scale quantum processors, one needs to envisage ways to control and readout arrays of thousands of quantum bits without using excessively large number of external connections. This is to prevent the introduction of noise, heat loads and decoherence mechanisms that would corrupt the quantum computation.
We are part of a consortium working across industry and academia devoted to developing a cryogenic chip for integrated readout and control of qubits entirely manufactured in a standard CMOS foundry.
Funding:
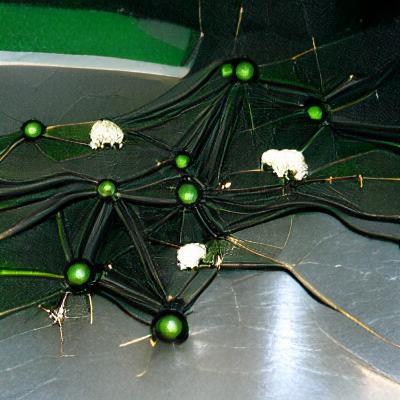
Autonomous Quantum Technologies (AUTOQT)
Quantum devices are fragile and easily deviate from their designed properties. Even creating two-level systems that constitute qubits is a challenge at scale due to non-idealities in the hardware materials. Consequently, complicated tune up routines, executed manually by highly trained researchers, are needed for the qubits to be useful. While this approach works for a handful of qubits, it is not scalable, hampering the development of commercial quantum computers. Similar problems beset other quantum technologies where operational stability goes hand in hand with scalability, such as precision metrology.
We are part of a consortium working across industry and academia that aims to develop machine-learning algorithms that will calibrate quantum devices autonomously and at the click of a button.
Funding: